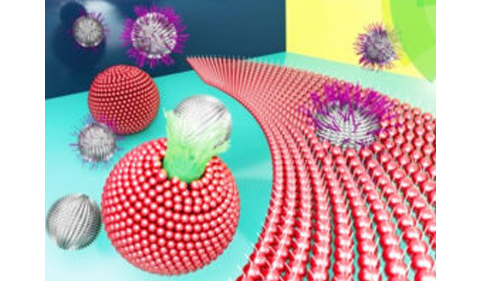
Diagram of monolayer and bilayer memberanes, the former is represented as a flat plane and the latter as a spherical structure (Illustration: Environmental Science: Nano)
By Amanda Biederman
NQPI editorial intern
Like the outermost walls surrounding a castle, the plasma membrane is the cell’s first defense against invaders. Biological membranes are semipermeable, meaning that they are designed to block unwanted molecules while permitting the passage of compounds necessary for cellular function.
And yet, an occasional intruder will slip through. Cytotoxins can invade living cells, potentially incurring cellular damage. Many researchers are interested in what makes these molecules toxic to organisms.
Chemical & Biomolecular Engineering Assistant Professor and Nanoscale and Quantum Phenomena Institute member Amir Farnoud is characterizing which types of compounds are likely to bind to the membrane. His recent work, conducted in collaboration with Chemistry & Biochemistry Assistant Professor and NQPI member Katherine Cimatu, was featured on the front cover of the February issue of Environmental Science: Nano.
Researchers construct synthetic models to study the properties of biological membranes, Farnoud said. But not all synthetic membranes are created equal. Many researchers study simple lipid monolayers (single layer), despite the fact that biological membranes in nature are actually bilayers (double layer).
In this paper, Farnoud investigated the applicability of synthetic monolayers to biological systems. His team, which included graduate student Saeed Nazemidashtarjandi and recent graduate Ali Asghari Adib, compared the binding of nanoparticles to both monolayer sheets and bilayer vesicles. They introduced silica nanoparticles with different functional groups (plain, amine, and polyethylene glycol) to both types of membranes.
“People use silica nanoparticles both for drug delivery and for industrial products,” Farnoud said. “This is relevant from a drug delivery standpoint (as well as) an environmental health hazard standpoint, because you can get exposed to particles in both ways.”
What they found was remarkable; some groups disrupted the monolayer exclusively, while the others disrupted only the bilayer. Farnoud said the difference is likely due to geometry; lipid monolayers are flat sheets, while bilayers (both natural and synthetic) are spherical. Synthetic bilayers better represent the dynamics of living cells and are thus likely a more appropriate model for biological systems.
Farnoud’s group is continuing its work with silica nanoparticles. He said he plans to design bilayers that are more complex by incorporating more diverse lipids that are characteristic of biological membranes. Additionally, Farnoud is interested in how rigid “lipid rafts” in the membrane influence these interactions.
“We’re making our membrane models much more sophisticated,” Farnoud said. “We’re trying to mimic the cell with our membrane model.”
This article first appeared in the Nanoscale and Quantum Phenomena Institute 2018 Spring Newsletter #19.
Comments