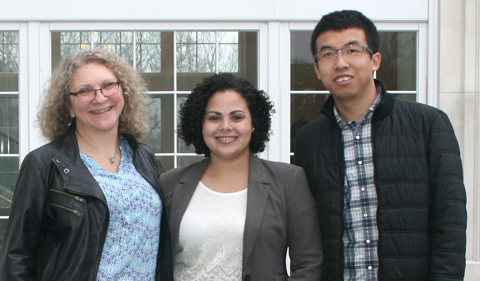
Drs. Nancy Sandler and Daiara Faria and doctoral student Dawei Zhai
New insight into flexible material one atom thick will help scientists to untangle key intrinsic properties of graphene not easily understood until now.
Graphene, a crystalline slice of graphite (a form of carbon), is perhaps one of the most important materials being studied worldwide.
Ohio University Associate Professor Dr. Nancy Sandler and doctoral student Dawei Zhai co-authored a paper with colleague Daiara Faria and others in the American Chemical Society journal Nano Letters titled “Tuning the pseudospin polarization of graphene by a pseudo-magnetic field.”
Sandler is a faculty member and theoretical physicist in Physics & Astronomy. Her publication contains results from the collaboration with an experimental group in Germany led by Dr. Markus Morgenstern at RWTH Aachen University, in Aachen.
“A single layer of graphene at the atomic level is so flimsy that even the most powerful microscope may introduce mechanical deformations altering its electronic properties,” Sandler says. “We provide the theoretical interpretation for the experimental data and show that when imaging a graphene membrane with a scanning tunneling microscope (STM), the membrane gets deformed, changing the expected STM patterns. The proposed models provide quantitative predictions for the changes in the electronic properties due to specific deformations and are corroborated by experimental measurements.”
Understanding the cause of the deformation phenomena gives scientists the opportunity to control it and eventually manipulate it for experimental purposes. For example, scientists may begin to manipulate the distribution of electronic charges depending on their experiment objectives, such as to improve the efficiency of a device application such as a sensor, or detecting impurities in a graphene sample.
An International Collaboration Started at a Workshop
Sandler’s contribution began in 2013 when she was on sabbatical in Berlin, Germany. The collaboration started at a workshop at Freie Universitat, Berlin where Morgenstern showed data and provided an interpretation. Sandler and her Brazilian colleague Faria wanted to verify his conclusion by providing a calculation. Faria is a professor at Rio de Janeiro State University (UERJ) in Brazil.
Sandler and Faria approached Morgenstern during a break and asked follow-up questions regarding his interpretation of the data. The two set out to verify the results using an equation to show how a mechanical deformation occurs at the atomic level. They shared their calculations with Morgenstern who invited them to collaborate in further studies.
The paper’s co-authors hail from Berlin, Germany, Rio de Janeiro, Brazil, as well as Manchester, United Kingdom, and Ensenada, Mexico. The international research effort succeeded due in part to discussions via weekly Skype meetings for almost two years. One of the co-authors is Konstantin Novoselov, a Russian-British physicist at the University of Manchester. His work on graphene with Dr. Andre Geim (also at the University of Manchester) earned them the Nobel Prize in Physics in 2010.
After the sabbatical, Sandler and Faria were awarded a travel grant by the American Physical Society to bring Faria of to Ohio University’s Athens campus for six weeks as a visiting scholar. Sandler and Faria are currently focused on understanding new experimental results from the research group led by Dr. Eva Andrei at Rutgers University on wrinkled graphene samples.
“International collaborations are important to bring different perspectives to subtle problems such as these,” explains Faria. “We have been collaborating for several years now and I am a professor at UERJ in part due to our successful research. Besides, my visits to Ohio University make it possible to have deeper conversations with first rate experimentalists and theorists working on graphene. The whole experience is very enriching.”
New Insight Opens the Door to Further Study Graphene Crystals
“The idea of controlling charge distributions with strain is an old one,” Sandler explains. “This has been done in the past by applying strain such as compression or stretching to a crystal in order to change some of its properties, say for example how well they reflect or transmit light, or if they change the polarization of light. So, the idea is not new.”
What is new is how this mechanism works for graphene that is a surface and not a three-dimensional crystal. The analogy would be to try to compress or stretch one sheet of paper versus a whole thick notebook. A sheet of paper may fold immediately as response to strain, but to get the same with the notebook much more stress is needed.
“What makes graphene different from all the rest of the materials we have at our disposal at the monatomic level,” Sandler says, “is that graphene is a very good conductor, where charges could move quite freely. The challenge here is how to make the charges more ‘static’ or somehow control their flow. This is a property that materials used in electronic industry have naturally—the so called semiconductors—but not graphene. So now we know how it works in graphene, too.”
Sandler points to other ways to use graphene, such as to suspend it. What she and her collaborators showed was that taking a “picture” of it with the STM makes it move around. The STM takes “pictures” by sending a current from the apparatus to the graphene sample below. Their results show how much current is needed to send when taking the “picture’ to produce a particular deformation.
Practically speaking, the insight allows for the design of specific deformations, for example one in which the charge accumulates in a pattern that resembles really thin, long wires and with them a circuit at the atomic level.
“Now that we know what type of effect a deformation has in the STM images, we can identify how much strain there is on a flake directly looking at the STM image without the need of doing further studies,” says Sandler. “There is a lot of interest in depositing graphene on different substrates that are semiconductors to gain different functionalities.”
A different substrate is basically another crystalline lattice where the atoms are positioned at different distances than the carbon atoms are in graphene. This “lattice mismatch,” as it is known, is enough to introduce strain in graphene that produces a re-accommodation of their electronic charges that are the only ones that move around.
“If we are going to make a device with the ‘complex structure,’ then it is important to know what happens with these free moving charges when we put graphene on top of a different material.”
Read the abstract and paper.
Comments